Overall scientific goals
The overall scientific goal of the lab is to understand how fundamental evolutionary processes shape the ecology and cellular physiology of microbial communities, and how the feedback from ecology and physiology provide constraints for further evolutionary processes.
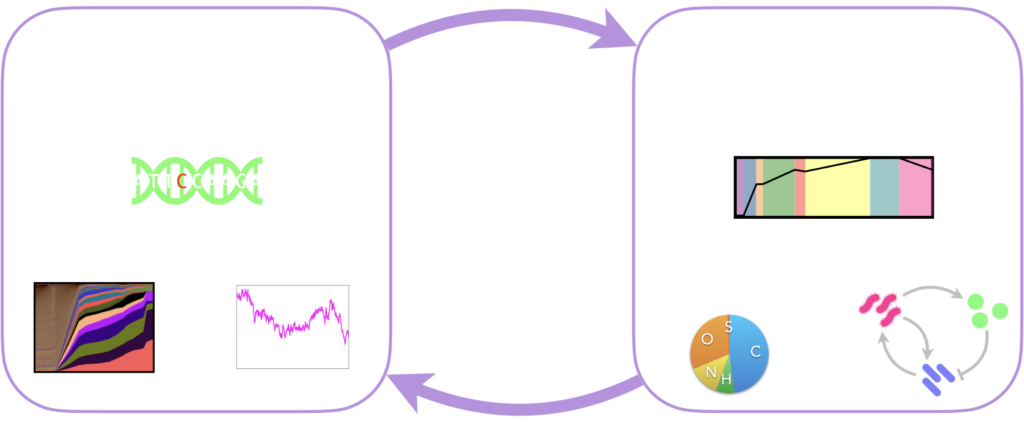
What are the fundamental processes of evolution?
Mutation. Mutation provides the raw variation for evolutionary change. One of our major hypotheses is that the supply of mutations significantly shapes the evolutionary outcome of a microbial community, through pleiotropy and diminishing returns. Therefore we are working to understand the mutation supply through a combination of laboratory experiments and modeling.
Examples from our work:
- Supply of de novo mutations vs. standing variation mediates different adaptation speeds under different antibiotics (Jasinska et al. 2020)
- Correlated mutation effects on growth rate and lag time produce correlations in the evolved traits (Lin et al. 2020)
- Mutations that reduce adenylate kinase function affect lag time more than growth rate (Adkar et al. 2017)
Selection. We want to understand how selection amplifies differences in physiological and ecological traits. We are especially interested in how selection acts on multiple traits simultaneously (performing multi-objective optimization), as well as its environmental dependence.
Examples from our work:
- Selection on multiple growth phases is approximately additive (Manhart et al. 2018, Manhart and Shakhnovich 2018, Fink et al. 2022)
- Selection can be non-transitive and frequency-dependent with variation across growth phases (Manhart et al. 2018, Manhart and Shakhnovich 2018)
Genetic drift. Random fluctuations due to finite-sized populations (genetic drift) limit the efficacy of selection. We are interested in how these adaptive limits posed by genetic drift shape physiological and ecological traits of microbes.
Examples from our work:
- Genetic drift prevents nutrient affinity from evolving arbitrarily high, and in some cases may decouple affinity from the nutrient’s environmental concentration (Fink et al. 2022)
What are physiological and ecological traits of microbial communities?
Growth dynamics. Microbes do not grow at steady state all the time, but are frequently switching between different growth states as their internal physiology and environment change. We want to know what these dynamics look like, especially in natural environments. One of our major hypotheses is that microbial populations spend little of their time in steady state, and therefore their evolution is driven mainly by selection on traits governing their transitions between states (e.g., acceleration and deceleration rates).
Examples from our work:
- Tradeoffs in traits of growth dynamics enable multiple species to form multistable communities (Manhart et al. 2018, Manhart and Shakhnovich 2018)
Resource consumption. Microbes consume not just one but many nutrients to survive and grow, including sources of carbon, nitrogen, phosphorus, and trace metals. One of our major hypotheses is that microbes are often in states of colimitation, where more than one of these nutrients simultaneously limits growth. We want to understand how evolution shapes these limitation states, and what are their consequences for physiology (such as proteome allocation) and ecology (such as resilience to invasions).
Examples from our work:
- Genetic drift can produce a mismatch between resource limitation and the environmental concentration of that resource (Fink et al. 2022)
- Microbes evolve toward simultaneous limitation of multiple resources (forthcoming)
Interactions. Since microbes live in dense communities of many different species, they are constantly interacting in both positive and negative ways. We want to understand how to quantify these interactions and how they shape, and are shaped by, evolution.
Examples from our work:
- Spatial structure facilitates evolution of stronger positive interactions (Gorter et al. 2020)
- Adaptation of resource limitation weakens positive interactions in cross-feeding communities (forthcoming)
- Cross-feeding interactions modulate effects of mutations in a microbial community (forthcoming)